Chapter I – Phosphorescence Produced by Light
1. The Different Forms of Phosphorescence
The name of phosphorescence is given to the property which several bodies possess of becoming luminous after having been exposed to various influences, that of solar radiations especially.
Phosphorescence, one of the most difficult problems in physics, and one of these of which the interpretation is the most complicated, realizes the apparent paradox of generating cold light — that is to say, light without any rise in temperature. In all our ordinary sources of lighting, light is only manifested after the bodies producing it have been first brought to a high temperature.
For a long time, phosphorescence was thought a phenomenon as rare in the mineral as in the animal world. Recent researches on deep-sea animals prove that, for an immense number of beings, phosphorescence is a normal means of lighting which enables them to guide themselves in those dark abysses of the sea where the sun never penetrates. It may be asked at the present day whether the animals knowing no other light but phosphorescence are not more numerous than those whose light is the sun. The phenomena of phosphorescence which formerly struck us by their exceptional character, now do so by their frequency.
We have often come across the action of phosphorescence in the course of our researches. The documents already published, which were confined almost entirely to the researches of Edmond Becquerel 50 years ago, not enabling me to explain the phenomena observed, I was led to take up this study anew. The new facts which I recognized are soon stated.
The customary divisions of phosphorescence being very artificial, it would be useless to reproduce them. I shall confine myself to dividing the phenomena into four classes. The three first have long been known. The fourth is due to my researches.
(1) Phosphorescence generated by light ~ (2) Phosphorescence independent of light and determined by different physical excitants such as heat, friction, electricity, and x-rays ~ (3) Phosphorescence by chemical reaction. In this class are placed the luminous phenomena exhibited by certain living beings ~ (4) Invisible phosphorescence. This comprises the production of light incapable of impressing the eye, but able to impress the photographic plate and to be rendered visible by different means.
We will only study, in this chapter, the phosphorescence produced by light.
2. Action of the Different Regions of the Spectrum on Bodies Capable of Phosphorescence
Many bodies, either natural, like the diamond, apatite, fluorite, and leucophane, or artificial, like the sulfides of the alkaline earths, have the property of shining in the dark after having been exposed for a moment to daylight.
With the exception of the diamond, of which the luminosity is, at times, very vivid, the phosphorescence acquired by minerals is always much inferior to that of artificially manufactured substances. A very large number of bodies are capable, as Becquerel showed with his phosphoroscope, of acquiring the property of shining for a small fraction of a second after being exposed to light.
In this last class of brief phosphorescence are placed the compounds called fluorescent. It was formerly wished to make of these a special class, on the pretext that they had the property of transforming invisible uv light into visible light. This property is really common to the various phosphorescent bodies. Nearly all, in fact, are capable of being illuminated by the uv end of the spectrum.
The action of the various regions of the spectrum is best observed with the phosphorescent sulfides. These sulfides are only four in number: those of calcium, barium, strontium and zinc. Exposed to the light for a few seconds, they acquire a phosphorescence which lasts several hours after the insolation [exposure to the sun], but constantly decreases during this period.
The great sensitiveness of these bodies to light comes very near to that of the gelatino-bromide photographic plates.
The sulfides of calcium, of strontium, and of barium have very similar properties; they hardly differ from each other, except by the color of their phosphorescence and the rapidity with which they are impressed by light. The sulfide of calcium is the most rapidly impressed, that of strontium the least so. This last requires several seconds of insolation to reach its maximum of illumination, while the sulfide of calcium can be impressed in 1/30 second in the sun.
The sulfide of zinc with green phosphorescence — and not those with the yellow or orange — possesses, for the study of the radiations from green to very far in the infrared, a special sensitiveness lacking in the other sulfides. Thanks to this, I was able to demonstrate the great transparency to light of bodies formerly deemed very opaque. A screen of zinc sulfide exposed to daylight when exposed to yellow, green, red, and especially to infrared radiations, is immediately extinguished. By precise measurements effected by diaphragms, of which I had measured the speed with a registering chronograph, I was able to note that, in less than 1/10 second, insolated zinc sulfide began to be extinguished in the infrared. I made use of this property to obtain instantaneous photographs in complete darkness, as will be seen in another chapter.
The extreme sensitiveness of zinc sulfide renders its illumination very variable, according to the proportion of infrared rays contained in the various sources of light. While the other sulfides become illuminated by the simple light of a paraffin lamp, zinc sulfide not only does not do so, but becomes immediately extinguished if it has been previously exposed to daylight.
The sulfides of calcium and strontium being little sensitive to the infrared rays, light up very well, on the contrary, with the light of a lamp or even of a candle. We thus obtain at once the means of distinguishing zinc sulfide if it be fraudulently substituted for zinc sulfide, although its phosphorescence possesses the same green tint. A screen of strontium sulfide becomes illuminated by the light of a candle; a screen of zinc sulfide previously exposed to sunlight, on the contrary, becomes extinguished. I must add that zinc sulfide with green phosphorescence, the only one serviceable for these experiments, is rarely met with in commerce.
If zinc sulfide is a valuable reagent for the infrared rays, calcium sulfide is as valuable for the radiations of the other extremity of the spectrum — that is to say, for the blue and violet rays. One-tenth second’s exposure to light suffices for its impression to commence.
To study the action of the various rays of the spectrum on phosphorescence, we have only to place in the focus of a spectroscope adapted for projection and mounted on a camera obscura, a plate covered with a phosphorescent sulfide. And after exposure to light to open the carrier in the dark.
I used a 3-prism spectroscope in front of which was a condensing lens, which is necessary in many experiments in order to increase the intensity of the luminous rays falling on the plate. In studying the action of light on phosphorescent bodies, these latter ought to be spread on glass or cardboard screens. The process of making phosphorescent screens, which I finally adopted after trying several, is the following: The sulfide is ground in an agate mortar and passed through a sieve of the very finest silk. It is then thoroughly mixed in the same mortar with a varnish known to color merchants a s bronzing varnish; the proportion of the powder added to the varnish may vary according to the quality of the later, but must not be less than 30 percent. When the mixture is perfectly homogeneous, it is poured, without being allowed to settle and in the same manner as collodion, onto a piece of cardboard laid flat. Care must be taken to gum on the edges of the card a small mount half a millimeter thick, and two or three mm wide.
If the mixture is poured onto a strip of glass, we obtain a transparent screen having exactly the appearance of fine ground glass. If the liquid is poured on cardboard, the screen is naturally opaque, but as the coating can be made thicker, it is more luminous. When too much varnish has not been put into the mixture, the screen is dry and ready for use in 15 minutes.
If we wish to preserve the image of the sulfide impressed by the various rays, all that is needed is to lay the screen for a few minutes on a photographic plate, which is afterwards developed in the ordinary way.
When operating with screens as above described and placed in the focus of the spectroscope nothing is more simple than to observe the action of the various parts of the spectrum. We then remark that the only part of the solar spectrum able to impress phosphorescent bodies starts from the blue to end very far in the uv. The rest of the spectrum — that is to say, all the rays from the green to far on in the infrared — are not only without action on the production of phosphorescence, but destroy it when directed on a phosphorescent body made luminous by the uv rays.
These facts had been summarily observed long ago, but the interpretation of them was very incomplete, for the excitation of light was attributed to a caloric excitement which obliged the sulfide to emit its phosphorescence in a short space of time. As far as concerns zinc sulfide especially, comparative experiments made on screens placed one beside the other have shown me that no period of excitement ever precedes the period of extinction. It is only in the case of calcium sulfide that a very slight excitement of phosphorescence is seen to precede its extinction.
From the point of view of its action on phosphorescent bodies, the solar spectrum thus comprises two regions very different, since their properties are quite opposed to each other: (1) a region of illumination corresponding to about half of the visible spectrum, ranging from the blue to the uv; (2) a region of extinction corresponding to the other part of the spectrum and going far into the infrared.
In the part intermediate between these two regions, varying slightly according to the sulfide used, but oscillating round the line F, there is a zone at once extinguishing and illuminating according to circumstances. With a screen of unexposed sulfide, it excites a certain degree of phosphorescence, which is always very slight. With a screen rendered very brilliant by previous insolation, it also restored the phosphorescence to a slight degree.
These last experiments, easy to observe in a camera obscura furnished with a spectroscope, are more conveniently, though less exactly effected, with a simple yellow glass. If, in a photographic slide closed y a light yellow glass, a screen of unexposed calcium sulfide is placed, and beside it another screen previously insolated, and the frame is opened in the dark after the exposure of the two screens to sunlight through the yellow glass, it is observed that they are both very slightly luminous. On the non-insolated one, the rays which are both extinguishers and illuminators have acted as the latter, and have produced a slight phosphorescence. On the screen previously insolated of which the phosphorescence was at first very bright, they have acted as extinguishers and have reduced it considerably. There therefore exist rays possessing the property of both producing a certain degree of phosphorescence and extinguishing it when it exceeds that degree.
The above results are of great importance. They will permit us, in another chapter, to resolve by very simple experiments the question of the existence of the antagonistic action of the two extremities of the spectrum. This point had been discussed for more than 50 years, and photographic experiments have not hitherto thrown much light upon it.
3. Phosphorescence of the Diamond
Although by its phosphorescent actions the diamond resembles the bodies we have just studied, it yet possesses special and highly interesting properties, the study of which delayed me sometime. It is for this reason that I devote a section to it.
The diamond differs from the other natural minerals capable of phosphorescence, because while in these last the aptitude for becoming luminous under the influence of light is in part destroyed by heat, in the diamond it is not so.
Although the property possessed by the diamond of becoming luminous in the dark after having been exposed to the light was known throughout antiquity, its phosphorescence has not been the object of any special study. Mineralogists had not even taken the trouble to seek for the origin of phosphorescent diamonds, and to note that while certain mines furnish diamonds which are always phosphorescent, the contrary is the case with others.
Cape diamonds, often as colorless as those of Brazil, and sometimes larger, are always very inferior to the Brazilian, not only by their hardness but also by their brightness. Placed by the side of the Brazilian, they appear dull.
In order not to confuse the particular with the general, I studied about 200 diamonds of all sizes, some Cape and some Brazilian. The latter mostly came from the Bahia mine, and presented all known varieties of color.
In order to study the visible phosphorescence, the diamonds were subjected to an illumination produced by a ribbon of magnesium 15 cm long, et on fire by a spirit lamp. This operation must always be effected by an assistant, while the observer remains in the dark so as not to be dazzled by the strong light of the burning magnesium, which prevents him afterwards from seeing the phosphorescence.
The first trials made on a parcel of about 100 diamonds of all tints, half of them Brazilian and the other half Cape, immediately showed me one curious fact. Nearly all the Brazilian and all those of the Bahia mine were, during the operation, brightly phosphorescent, as much so as an isolated fragment of zinc sulfide. Not one of the Cape diamonds was phosphorescent.
The non- phosphorescence of the Cape diamond is, however, not absolute, for after one has remained in the dark for at least 20 minutes, in order to rest the eyes — during which time the diamonds should be exposed to the sun by an assistant — a very slight phosphorescence on nearly half of them is detected. This phosphorescence is on the border of the perceptible minimum of light, and is in no way comparable to the brilliant light of the Brazilian diamonds.
The same experiments repeated many times with other diamonds of known origin have always afforded the same results (1).
[(1) This means of distinguishing Brazilian from Cape diamonds is very exact, and has ore than once enabled me to enlighten purchasers of the real value of their diamonds, indications which have always been found correct by appraisers. I was able to discover immediately, out of a parcel of 60 Cape diamonds, a Brazilian one placed there in error. This diagnosis is available to everyone, and will enable many persons to observe that they sometimes pay nearly double their value for diamonds. A diamond sold for 10,000 francs on the strength of it being Brazilian is in reality only worth 6000 francs if it is from the Cape.)
As a first conclusion, we see that it is not to the coloration of the diamond, but to its geological origin, that its phosphorescence is due. Whitish or yellowish diamonds from Bahia are phosphorescent, while those from the Cape are not, whatever be their color.
As with the various phosphorescent bodies, pulverization notably reduced the phosphorescence of the diamond, but does not destroy it.
All diamonds which phosphoresce by the action of light also do so when a pencil of x-rays is directed upon them.
Subjected to the electric induction spark in the method described in another chapter, all diamonds become phosphorescent. They likewise become so when exposed to the influence of radium, even through a thin leaf of aluminum.
We shall see in another chapter that diamonds likewise exhibit the phenomenon of invisible phosphorescence.
The aptitude of diamonds for becoming phosphorescent by the action of light is not destroyed by heat, as in the case with many mineral substances. While, after being calcined for 15 hours, it was destroyed in the case of many bodies, such as fluorspar, apatite, etc., yet I have been able to heat diamonds to 1000° C for 60 hours without altering their aptitude for phosphorescence. They have then been reduced to an impalpable powder in an agate mortar, and then calcined over again (1). This has not prevented them from again shining after insolation.
[(1) This operation was necessary in order to discover if the phosphorescence were not due, in the case of the amethyst, so foreign bodies destructible by heat, It is not a very economical one, for the mercantile value of cut diamonds of small dimensions is about 1300 francs per gram. This cost would be much reduced by employing rough diamonds, but Brazilian rough diamonds are almost undiscoverable in Paris, merchants finding greater profit in importing them cut.)
This persistence of the aptitude for phosphorescence, notwithstanding so prolonged a calcinations, shows that, if the phosphorescence of diamonds is due to the presence of foreign bodies, these bodies are not altered by heat, or at all events by a heat below that at which the diamond is destroyed.
We are generally taught in the textbooks that the rough diamond is not phosphorescent, and only acquires that property after being polished. Landrin, in his Dictionnaire de Mineralogie, expresses himself as follows: — “A crystal of fluorite is only phosphorescent when polished. The contrary is the case with the diamond, which only gives light after having been subjected to polishing, and does not manifest this faculty when in its natural crystalline state”.
I was almost convinced of the inexactitude of this assertion, since, according to my observations, the pulverized diamond does not lose its aptitude for phosphorescence. I was anxious, however, to verify by experiments this belief of mineralogist; for the scientific consequences of the property they attributed to the polished diamond would have been very great. As I expected, it was one of those classic errors repeated without verification to which repetition at length gives indisputable authority. Having succeeded in procuring some rough diamonds from Brazil, yellow, blue, and transparent — some crystallized, others rounded — I was enabled to note their phosphorescence by the action of light.
The phosphorescence of diamonds seems to be connected, as in the case with other bodies, especially the sulfides before mentioned, with the presence of traces of foreign substances. The most transparent diamonds, when incinerated, leave a small quantity of ash hardly less than 2 percent, containing various bodies — magnesia, lime, and especially iron.
4. Relation Between the Intensity of the Phosphorescence and the Temperature of Insolated Substances
Is the degree of phosphorescence which an insolated body may reach, closely related to the temperature of this body during insolation? Experiment alone can decide this question.
At a temperature markedly below 0° C, and varying according to the substance, bodies exposed to the light do not acquire visible phosphorescence. Above this temperature the intensity of phosphorescence obtained by a body exposed to the light increases when heated up to 100° C. Above 100° C, the phosphorescence it may acquire diminishes, and towards 500° C falls to nothing. The influence of this high temperature may be explained by admitting that the precipitation of the light by heat is then as rapid as the absorption. The expulsion taking place at the same time as the absorption, the phosphorescence does not appear.
The above facts can be verified by means of screens of calcium sulfide placed on cards divided into halves. The two halves, placed side by side in the dark, on recipients brought to different temperatures, are examined as soon as they have been lighted up by a magnesium ribbon. Towards about -190° C, which temperature is obtained by plunging the screens of sulfide into liquid air, no phosphorescence under the action of light is observed, as was first recognized by Dewar; but on withdrawing the screen, and leaving it for a moment in the dark at the ambient temperature, it becomes luminous. The passage from -190° C to the ambient temperature represents to the sulfide a considerable increase of heat, which causes it to rapidly expel the invisible phosphorescence acquired at -190° C.
But it is between 0° C and 100° C that the experiment is most easily effected. One half of the screen having been placed on a block of ice, the other on a sand bath heated to different temperatures, we observe after exposure to magnesium light that the sulfide exposed when heated to 100° C is much more brilliant than that exposed to 0° C. In the case of calcium sulfide the difference is considerable, but much less so in the case of zinc sulfide.
If one of the two screens is placed on a plate heated to 200° C, and the other kept at the temperature of the ambient air, i.e., about 15° C, we recognize, after illumination by magnesium, that the screen heated to 200° C is much less brilliant than the other. Repeating the same experiment with a screen heated to 500° C, we only obtain on it an extremely light phosphorescence. I have given above the probable reason for these differences.
5. Loss of phosphorescence by the Action of Time
What has been said of the action of various rays of the spectrum and of the temperature during exposure has already proved that the intensity of phosphorescence depends on several factors.
There remains to us one more to study, viz. Time. All authors have regarded it as having a preponderating influence.
Time has, on the loss of phosphorescence, an influence evident but inferior to that of temperature. As the visible phosphorescence which follows insolation hardly exceeds for the most sensitive bodies the duration of a few hours, time has hitherto been considered as the principle element destructive of phosphorescence. This element has in reality no fundamental influence, since, by means of a suitable temperature, we can entirely eliminate its action.
Curves of the loss of phosphorescence as a function of the time have several times been published, and their equations calculated. These curves would only mean something if they expressed the loss at a given temperature, for this loss varies with the temperature. The number of curves would then have to be very considerable, since a special one would be required for each temperature.
Curves thus constructed would have, moreover, no characteristic in common. At a very low temperature varying with each body, the curve of loss as a function of the time would be represented by a line nearly horizontal, which would signify that at that temperature the phosphorescence diminished very slowly. At a high temperature the curve would be almost vertical, signifying that the loss of phosphorescence is, on the contrary, very rapid. At an intermediate temperature the curve would be represented by a line at first and during the first few seconds after insolation almost vertical, and then after a fairly short time almost horizontal.
If the influence of temperature had been more carefully studied, it would have been seen long ago that these curves of the loss of phosphorescence as a function of the time, since they set aside a much more important factor than time, could possess no correctness.
Not only is time a secondary factor in the phenomenon, but a moment arrives when it has no effect on the loss of phosphorescence. We shall see, in fact, that after a certain emission of light at a given temperature, the body retains indefinitely a residual phosphorescence so long as its temperature is not again raised.
This law is very general. The loss of phosphorescence after insolation, which appears to be spontaneous in certain bodies, such as the sulfides, is the result of their not being able to retain at the ordinary temperature an excess of phosphorescence, against which an antagonistic force, to which I shall refer later on, contends. By cooling them sufficiently, we suppress all emission. They are then analogous to bodies such as fluorite or apatite, which are only phosphorescent above 50° C, and on which time has absolutely no effect.
The action of temperature is therefore much more important than that of time. It is this, and not time, which regulates the emission of phosphorescence.
There can, however, be deduced some useful information from the study of the loss of light under the influence of time if the temperature be kept constant for the whole duration of the phosphorescent emission.
Let us take a screen of insolated calcium sulfide, keep it at 15° C, and examine the curve of the loss of its light. It will fully justify what has been said regarding the part played by temperature.
If this last remains constant, the fall of the curve, representing the loss of phosphorescence as a function of the time, is for the first minute after the insolation almost vertical. It then bends slowly, becomes less and less oblique, and finally quite horizontal. At this moment the body no longer radiates, time no longer influences it, and it keeps intact an invisible provision of luminous energy which it will part with only by various means, or, more particularly, by a rise in its temperature. We will return at length to this last point in another chapter.
The general appearance of the above curve shows, indeed, that things happen as if the reaction exciting the phosphorescence placed itself in equilibrium with an opposing force acting in a converse direction. Immediately after its insolation the screen contains an excess of phosphorescence. Under the influence of the opposing force, this excess is dissipated, rapidly at first, then slowly when that moment approaches when the equilibrium is established between the phosphorescence and the opposing force. When this equilibrium is attained, the reactions which produce the phosphorescence stop entirely. The opposing force being unable to act further on the reaction which generated the phosphorescence, the body will retain its residue of phosphorescence until a rise in temperature again destroys the equilibrium.
I do not know of what this opposing force consists. I can only say that things take place exactly as if it existed. This interpretation has also led me to the discovery of the discovery of the phenomena of invisible phosphorescence studied in a future chapter.
Chapter II – Phosphorescence Produced by Heat
1. Method of Observation
Many minerals in nature possess the property of acquiring a bright phosphorescence when brought to a low temperature without first having first been exposed to light. If the heating is sufficiently prolonged, they lose all their provision of phosphorescence, and no longer shine when heated after being allowed to cool. They regain, but in a slight degree, the property of shining by a rise in temperature, when we expose them to the light after having exhausted their phosphorescence by heating.
These facts, which have been known for a long time, represent about all that is to be found in textbooks on physics regarding phosphorescence produced by heat.
It is astonishing that so surprising a phenomenon as the phosphorescence caused by slight heat should not long ago have engaged the attention of physicists. With the theories current it is not apparent in what form a body cold have preserved since its geological formation a provision of luminous energy which we can force it to expend by fringing it to a temperature which may often be less than 100° C.
The experiments now to be mentioned prove that the explanation of the phenomenon is much simpler than that last given. It is not in the form of luminous energy that bodies preserve for ages their aptitude for phosphorescence on a slight rise in temperature. They have simply preserved compounds incapable of combining with each other at an ordinary temperature, but able to do so when it is raised, which is the case with many chemical reactions. Phosphorescence simply arises from these combinations. Bodies in presence of each other which will not combine under a certain temperature, can evidently retain for an indefinite period the aptitude for phosphorescence just as chlorine and hydrogen remain inactive for an indefinite period in darkness, and combine only when we introduce some exciting agent such as light.
If I had not deemed it useless to modify accepted classifications, it is really in the paragraph devoted to phosphorescence by chemical reaction that I should have inserted that which concerns phosphorescence produced by heat.
The methodical study of the action of heat on phosphorescence demands a little apparatus easy to construct. It will serve us not only for study of the action of heat, but likewise for that of the infrared and of invisible phosphorescence, which we shall examine in other chapters.
The method of observation plays an important part in the research into the phenomena of phosphorescence by heat. The processes of observation employed by mineralogists, which consist in placing the bodies to be examined on metal plates or crucibles at a red heat, are quite primitive, and it is because their processes are rude that so many phenomena have escaped them.
The moment the source of heat becomes visible, whether it be produced by a simple spirit lamp, or a gas burner with a blue flame, the eye is dazzled, and all feeble phosphorescence escapes observation.
For the purpose of studying the phosphorescence produced near the regions of the red rays, I simply make use of a large metal box, without a cover, inverted over a small spirit lamp. Its sides should be serrated at the bottom to allow the passage of air, and, in the part over the lamp, an orifice made in which to place a small copper cup one-tenth of a millimeter thick, which receives the body to be heated. By reason of the thinness of its walls this cup can be heated and cooled almost instantaneously. It can be made red-hot with the greatest ease, whenever necessary.
We procure from a dealer in photographic apparatus one of those triple burner lamps with a metal chimney, which are used for lighting magic lanterns. On the upper extremity of the chimney, which is pierced with lateral holes for the passage of air, we place horizontally, in order to stop the light entirely and to have a plane surface to receive the bodies required to be heated, the lid of a biscuit box. The glass or mica plate through which the light of the lamp issues, and which slides in grooves, is replaced by a metal plate which thus masks all the visible rays. As a little light still issues from the lower part of the apparatus, the latter is surrounded by cardboard, which entirely envelopes it.
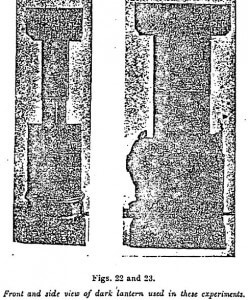
Thus constituted, the apparatus is all that is needed for researches where heat alone comes into play. But in many experiments on invisible phosphorescence, we need an abundant source of infrared variations of determinate wavelength. In order to obtain these, it suffices to replace the metal plate in front of the lamp by a strip of ebonite about 0.5 mm thick, fixed between two strips of glass to prevent its shape being altered by the heat. The ebonite may be replaced with advantage by black glass, but this must be of good quality, so that the disc of the sun cannot be seen through it, and yet that it may be transparent to the infrared. This being difficult to procure, I do not dwell on its use.
Under these conditions, the lamp gives the following temperatures: —
(1) With all three burners alight, about 225° C; (2) With two burners, about 130° C; (3) With one burner, about 65° C; (4) Temperature of the vertical side of the metallic chimney of the lamp, about 105° C.; (5) Temperature at 1 cm from the vertical side of the chimney, about 50° C.
In front of the strip of ebonite or black glass, the temperature at 2 mm of their surface is only 30° C., but through these bodies there passes a great quantity of invisible infrared radiations, of which a great part is comprised in the region of the spectrum from 0.3 microns to about 3 microns. It is these which act on phosphorescent substances, and possess the property of passing through opaque bodies, as we shall see in another chapter.
All the metal sides of the lamp also, of course, emit infrared rays, as do all heated bodies, but these radiations, having a wavelength of 5 microns to 10 microns, do not act on phosphorescent bodies.
In this manner is constituted the source of heat and of dark radiations which I have designated by the name of ‘dark lamp’.
The instrument should be placed in an absolutely dark room. It is preferable to make the experiments in the evening, for then the eye is much more sensitive to dim light. If the operations are effected in daylight, one should remain 15 minutes in the dark and let the illumination, whether by magnesium or by the sun, be carried out by an assistant, while the observer remains in the dark. This last precaution is quite indispensable. Many phenomena escaped me at the commencement of my experiments for want of this precaution.
2. The Properties of Bodies Phosphorescent by Heat
The list of bodies phosphorescent by heat is very long, though in treatises on mineralogy but a small number a re given: fluorite, fluorspar, topaz, several varieties of calcium phosphate, leucophane, diamond, are noticeable among them. A large number of others must now be added to these different bodies. Among those I have examined I will mention especially the Siberian and Bogotan emeralds, the Auvergne amethyst (but not that of Madagascar), the chlorospinel, the opal, cryolite, scheelite, Wagnerite, phenacite, petalite, castor, pollux, colemanite, talc, baryta, etc.
Most of these compounds are of aqueous origin. Bodies very phosphorescent by light — that is, the sulfides of the alkaline earths — only acquire, on the contrary, the property of becoming phosphorescent after having been subjected, during their manufacture, to a high temperature.
Many of the bodies phosphorescent by heat, and especially the Australian opal, leucophane, and the apatite of Estrmadura, together with nearly all the different varieties of fluorite, likewise become phosphorescent by exposure to light; but their phosphorescence, being in that case very slight, is only perceived by the observer after a stay of 15 minutes in the dark.
As the above bodies possess identical properties, and only differ from one another by the intensity of their phosphorescence, its duration, and the temperatures at which it manifests, I shall here confine myself to the examination of some of those which offer the brightest phosphorescence — such as the apatite of Estremadura and the green fluorite especially.
The apatite of Estremadura, a body easily procured in large quantities, is the one of which the phosphorescence commences at the lowest temperature. Reduced to powder, and put in a tube placed in a sand bath or in water slowly heated in the dark, it begins to shine with a feeble light at 51° C. Heated to 200° C it acquires a bright phosphorescence, which lasts for about an hour, on condition, be it understood, that that temperature is not exceeded. If brought to a red heat its brilliancy will be greater still, but will pass off in less than a minute.
The different varieties of fluorite noticed by me — and they are very numerous — behave much like apatite, but are always less luminous.
The temperature at which the fluorites become phosphorescent varies greatly with the different samples. I have examined some 30 of different origins, and have noted that the majority sine at temperatures below 200° C. The varieties which become illuminated at the lowest temperature are those from Morgen (Saone and Loire), from the Pyrenees, and from Schwarzenbergen in Saxony. They commence to shine at about 62° C.
It has been said that the transparent fluorites do not exhibit phosphorescence by heat, This erroneous assertion can only be due to an imperfect method of observation. All the fluorites that I have examined, including some which were colorless and transparent as glass, and which are used in the manufacture of prisms and lenses, are very phosphorescent by heat. I have, moreover, found but one sample of fluorite which was not phosphorescent by heat, i.e., the yellowish cubic variety in little crystals which comes from Herblay.
All fluorites are slightly phosphorescent by light, save the yellow variety above mentioned and a green crystallized fluorite coming from Durham.
When, after the expulsion by heat of all their phosphorescence, the bodies examined above are exposed to the sun and heated anew, they again shine, but much less brightly than the first time.
The property appertaining to several bodies like fluorite, of becoming phosphorescent at one and the same time by heat and by light, has been the origin of an error which for a century runs through all treatises on mineralogy, and has been religiously repeated by various authors, including Becquerel, without their ever having taken the trouble to verify the grounds of it. According to them, certain varieties of the green fluorite called chlorophane shine indefinitely in the dark at a temperature of 30° C — that is to say, they are luminous in darkness nearly all the summer in our climate and the whole year round in hot countries. This is how Boudant expresses himself with regard to this matter: —
“There are varieties designated under the name of Chlorophane, of which some are phosphorescent at the average temperature of our climate, so that they shine constantly in the dark, while others simply require the temperature of the hand” (Mineralogie, 2nd edition, vol. 1., p. 203).
The most recent and important German treatise on mineralogy, that of Naumann and Zirkel, expresses the same idea: “Many topazes, diamonds, and fluorites become phosphorescent at the temperature of the hand. The green fluorite (chlorophane) often remains brilliant for weeks after being insolated”.
Although this fact enunciated by mineralogists seemed theoretically very improbable, I desired, by reason of its consequences, to verify it. I therefore examined innumerable samples of the variety of fluorites called chlorophane from the most important firms of mineralogists in Europe. Not one shone at 30° C., nor at the temperature of the hand.
Evidently this was bound to be so. Suppose, in fact, they could have shone at from 30° to 37° C. Having been many times exposed to this temperature since their geological formation, they must have lost long ago their provision of phosphorescence, unless we class them in the category of radioactive bodies spontaneously and perpetually phosphorescent.
After having discovered that the fact set forth by physicists like Becquerel and mineralogists like Boudant was incorrect, the cause of the error had to be established. Its explanation was very simple.
The fluorites in question acquire, by insolation, a very feeble phosphorescence, which the eye, unless previously rested, does not perceive in the dark. If heated subsequently by holding them in the hand, it happens, as is also the case with all phosphorescent bodies, that their luminosity becomes more vivid. It is then easily seen, and the observer concludes that it is the heat of the hand which has produced this phosphorescence. To be convinced that it is nothing of the kind, we need only leave the sample in the dark for 48 hours. Then, placing it in the hollow of the hand, it is noticed that it no longer shines at all. If it be exposed to the light and then placed in the hand, it will shine at once. Consequently light is plainly the origin of the phenomenon.
The cause of the error, so faithfully reproduced in all treatises of mineralogy, was therefore that operators had left their specimens of fluorite exposed to the light before putting it in their hand. Far from shining all the summer, fluorite actually does not keep this property after insolation longer than ordinary phosphorescent bodies — that is to say, for a few hours.
What is the origin of the phosphorescence manifested by certain bodies under the influence of heat? Phosphorescent sulfides can retain indefinitely a part of the energy stored up by exposure to light. Might it not then be supposed that the phosphorescence emitted under the influence of heat is due to the fact that the bodies manifesting it have often been manipulated in the light before reaching the hands of the observer? Their phosphorescence would consequently be of recent origin, and analogous to that preserved for years by the sulfides after insolation, which can be made to appear by heating.
In order to solve this question, it was necessary to examine bodies which had not been brought to light since their geological formation.
For this purpose, it sufficed to break up, in absolute darkness, large blocks of apatite and fluorite, to extract fragments from the center of these blocks, and to subject them to the influence of heat. These fragments shone as vividly as those taken from the surface of the blocks. It was therefore not to a residuum derived from the recent origin of light that their phosphorescence was due.
When studying the causes of phosphorescence, we shall see that the light manifested by bodies under the influence of temperature is the result of chemical reaction provoked by heat. In bodies phosphorescent by light, in ordinary temperature, these combinations are easily reformed after being torn apart, and that is why all their phosphorescence is restored to them by exposing them anew to the light when they have become dark. For bodies which only become phosphorescent at a more or less elevated temperature, and only regain very feebly the aptitude for anew phosphorescence by heat after insolation, it is probable that the combinations destroyed by the temperature are only very incompletely reformed by light. We shall see, however, that they may be completely regenerated under the influence of electricity, and that there can be restored to bodies which have lost it, the property of shining anew under the influence of heat as vividly as the first time they were heated.
The aptitude of bodies to again become phosphorescent in the light after having been heated is, moreover, only lost with great difficulty. All those on which I have experimented — fluotire, leucophane, apatite, etc. — must be calcined at a red heat for 15 hours before losing it entirely. Ten hours of calcinations were quite insufficient.
How does a protracted rise in temperature act? Why does it take from a body its aptitude for giving phosphorescence?
The first idea which strikes one is that the calcinations destroys certain foreign bodies necessary to phosphorescence.
This hypothesis seems at first sight justified by the change in appearance undergone by a small number of bodies subjected to this calcination. The violet amethyst, when calcined, loses its color entirely, and becomes as transparent as glass. It has therefore evidently lost something which produced the phosphorescence, since this can no longer be restored to it afterwards by the action of light or heat.
But that which is true as regards amethysts and, perhaps, also other bodies, ceases entirely to be so for the great majority of phosphorescent substances. By calcining them for 15 hours, I have caused them to lose entirely their property of becoming again phosphorescent by heat after insolation. But by this operation I eliminated no foreign element. I simply destroyed the aptitude of the bodies present for chemical combination. Proof of this is furnished by the fact that by suitable treatment the aptitude to become phosphorescent by heat as they were before calcinations can be restored to calcined bodies.
To restore to a calcined body its aptitude for phosphorescence, it suffices to pass electric sparks through it for a certain length of time. This property of the electric spark was summarily observed as early as the first years of the last century. As, however, the induction coil was not then known, I thought it might be useful to take up again the study of the phenomenon.
The bodies subjected to the influence of the induction spark were placed in a glass tube closed at its two extremities by corks, through which passed copper rods connected to a strong coil. By approaching and withdrawing these two rods sparks of from 1 to 15 cm could be obtained at will.
The length of the spark has a manifest action. If, for example, calcium carbonate is electrified by a spark of 2 cm, it shines very feebly at 200° C. It shines, on the contrary, very vividly if the spark is 15 cm long.
The duration of the electrification has also a notable influence. If powdered glass is electrified for 5 seconds only, it shines but feebly at 200° C. It shines, on the contrary, vividly at that temperatures if the action of the spark is prolonged for 5 minutes.
In a general way, electricity simply vivifies the phosphorescence of bodies which, without its influence, would have been very weak, but it has never, to my knowledge, communicated it to bodies without any traces of it. In addition, it lowers the degree at which they begin to shine by the action of heat. Cape diamonds, hardly phosphorescent at 200° C., shine brilliantly at this temperature after the passage of electricity through them. Bologna spar, which only shines at 500° C. without electrification, is brilliant at 200° C, so soon as it has been electrified.
There exists indeed a very small number of bodies which present no trace of phosphorescence at 500° C., and give a very slight one at that temperature after electrification. This is, for example, the case with barium bromide. But then it may be supposed that before electrification they manifested a phosphorescence too slight to be visible. I found this conclusion on this general fact — that electrification only renders more vivid a slight phosphorescence. Even with bodies which possess a bright phosphorescence when they are impure, such as the sulfides of calcium, barium, strontium, and zinc, the electric spark confers on them no trace of aptitude for phosphorescence when they are pure.
The only bodies to which no phosphorescence can be restored by electricity are those to which calcinations really causes a loss. Such is the case with the amethyst, which loses its coloring matter when calcined. To all other bodies phosphorescent by heat, electrification restores the phosphorescence lost by calcinations. I have noted it especially in fluorite, leucophane, and apatite, which after having been calcined for 15 hours, lost the property of regaining phosphorescence by heat after insolation.
In this last case the body heated to 500° C will first emit all it can lose at all lower temperatures, and consequently will no longer be able to shine at less than 500° C. To give it back its phosphorescence, it will have to be heated again to 500° C or more.
This general law of the emission of phosphorescence as a function of the temperature, already pointed out in the last chapter, enables us to unite in one class certain bodies phosphorescent by light and heat, till now separated. We can very easily, in fact, transform bodies phosphorescent by light into bodies phosphorescent by heat. The sulfides of calcium, zinc, and strontium, kept in the dark for days or years after their insolation, are identical with bodies phosphorescent by heat, such as the amethyst, fluorite, leucophane, and apatite. It will suffice also to heat them up to about 70 C to enable us to see them shine.
The analogy may be, however, carried much further. The substances we call phosphorescent by heat only shine at determinate temperatures varying from to 300° C, according to the bodies observed. The luminous sulfides, which shine when insolated at the normal temperature differ from the preceding bodies solely because the temperature at which they cease shining is much lower. Dewar, as has been said, has shown that, in liquid air, insolated bodies acquire no phosphorescence, but that they manifest it so soon as they are allowed to return to the ambient temperature. To bring bodies refrigerated to about -190° C back to the temperature of the ambient air, simply signifies that we heat them to more than 200° C (1). Sulfides which are dark at -190° C and shine at 0° C are analogous to bodies which become phosphorescent by heat at a temperature of +200° C. Both kinds are only phosphorescent when they have been sufficiently heated. A phosphorescent sulfide kept for an indefinite time at -190° C would always remain dark, as would apatite kept indefinitely at a temperature lower than +50° C. The two bodies only differ, I repeat, by the temperature at which the combinations accompanying their phosphorescence take place.
[(1) These are, however, changes much too great. The divers phosphorescent sulfides cease to shine long before -190° C, and return to phosphorescence long before 0 C is reached. The temperature varies, moreover, with the various sulfides.]
When bodies phosphorescent by heat have been brought to the temperature at which their phosphorescence becomes extinguished, they cannot, as has been said, recover by exposure to the light more than a very small part of it. This is probably due to the fact that the chemical reaction which produces the phosphorescence can only be partially regenerated in certain bodies by light, while in others it is totally so, as especially with the phosphorescent sulfides and diamonds. There exists no real barrier between bodies phosphorescent by light and those phosphorescent by heat.
From the above consideration may be deduced the following laws, applicable to all bodies phosphorescent by light or by heat: —
(1) There are no bodies phosphorescent without heat. A body capable of being rendered phosphorescent by light will only manifest its phosphorescence at a certain temperature.
(2) For each phosphorescent body there is a minimum temperature below which exposure to the light cannot produce visible phosphorescence.
(3) To each temperature corresponds a certain emission of phosphorescence which cannot be exceeded.
(4) Bodies which heat renders phosphorescent also become so by light, but only slightly. They differ from those vividly phosphorescent by light only in that in these last the luminous rays entirely regenerate the whole of the destroyed phosphorescence instead of regenerating only a part of it.
Chapter III – Phosphorescence Proceeding From Causes Other Than Light and Heat
(1) Phosphorescence through Impact and by Friction
A large number of bodies become phosphorescent by friction or by impact. In the case of some — apatite, leucophane, diamonds, sugar and uranium — the impacts or movements may be slight; for others — such as fluorite and silex — the friction or impact must be somewhat energetic.
Whether violent or slight, they only generate a very weak rise in temperature, quite insufficient to bring them to incandescence. It cannot therefore be heat which produces the phosphorescence observed in these substances mentioned.
Neither does the shock not the friction determine the preliminary operations necessary to produce a reaction propagated from point to point, as in the decomposition of nitrogen iodide for instance. Phosphorescence by shock or friction does not, in fact, survive the disappearance of its cause, as in the case with that due to light.
The oxygen of the air plays no part in phosphorescence by shock, which is favored, on the contrary, by the total absence of oxygen — that is to say, by a vacuum. An exhausted tube containing a little mercury becomes luminous as soon as the metal is displaced by a slight movement.
Several bodies which friction renders phosphorescent also become so by the action of light and of heat. There exist, however, a small number such as metallic uranium, which are rendered phosphorescent exclusively by friction.
If, by a very prolonged calcinations, we destroy the property possessed by may bodies — leucite, apatite, and leucophane — of phosphorescence under the influence of heat, these substances regain the facility of becoming luminous by friction. We shall notice, on the other hand, that bodies hardly at all phosphorescent by heat, like Cape diamonds, become vividly so by friction.
The result of these experiments seems to be that the reactions which give rise to bodies an aptitude for luminous and caloric phosphorescence differ from those which cause them to shine after friction or a shock. It is probable, however, that it is an action of different causes producing the same effect.
2. Phosphorescence by X-rays, Cathode Rays and High-Frequency Effluves
The phenomena of phosphorescence from the above causes are very well known, and shall have very little to add to them.
We know that a large number of bodies, and among them compounds not phosphorescent by other processes, such as rubies, become extremely luminous when exposed to the bombardment of the rarified gases in a Crookes tube. The phosphorescence of those which become brilliant in a strong light, such as diamonds and certain sulfides, is greatly increased. It is sufficient to place them in a Geissler tube subjected to induction sparks. With some calcium sulfide in such a tube, I was able to obtain a phosphorescence of which the brilliancy, surface for surface, reached 7/10 of a candlepower.
The x-rays from a Crookes tube provoke only a very weak phosphorescence, and only in a small number of bodies, contrary to what is generally thought. Even with those phosphorescent in ordinary or in uv light, the illumination is slight/ The x-rays make calcium sulfide luminous to an extremely slight extent, much less than would a simple candle. They also illumine moderately — that is to say, much less than ordinary daylight — zinc sulfide, and herein differ entirely from the uv radiations to which it was for a long time sought to assimilate them.
On the fluorescent substances — that is to say, those of which the phosphorescence does not survive the cause producing it — the x-rays act, on the contrary, very vividly, therein behaving like uv rays, but more energetically. It was even, as is known, this property which caused their discovery, and rendered them utilizable. Platinocyanide of barium, of which radiographic screens are formed, is not the only salt which becomes strongly luminous under their action. Apatite, leucophane, fluorite, and, above all, Brazilian diamonds are also illuminated, though more feebly.
Many bodies, diamonds, zinc sulfide, etc., which are luminous under the influence of the x-rays, also become so under the action of the salts of radium. Cape diamonds, not phosphorescent by light, become so in presence of radium bromide, even when this salt is enclosed in a thin metal tube.
Sir W. Crookes has remarked that diamonds placed for several months in contact with radium, acquire such a radioactivity that it does not disappear when they are heated to a dark red.
Taken altogether, the preceding phenomena show us that certain bodies become phosphorescent from very different causes — light, shock, cathode rays, x-rays, etc.; these causes perhaps act, as has been said, by producing reactions, which, if not identical, are at least of the same order.
(3). Phosphorescence by Chemical Reaction
For a long time, the only chemical reaction known to produce phosphorescence was the slow oxidation of phosphorus. It is known, by recent investigations, that phosphorescence accompanies a considerable number of reactions.
Most of these, however, are badly defined and result from somewhat complex mixtures. Thus, for instance, those produced by mixing certain organic bodies, such as esculine or various essences with an alcoholic solution of potash, or, again, that obtained by pouring aluminum sulfate or gold chloride into an alkaline solution of pyrogallol (1).
[(1) For producing a rather lively phosphorescence there has been recently mentioned a mixture of: 10 cc 10% solution of pyrogallic acid, 20 cc 40% solution of potassium carbonate, 10 cc 30% solution of formol. The above mixture should become phosphorescent by adding to it 30% of concentrated oxygenated water.]
To arrive at a precise knowledge of the causes of phosphorescence by chemical reaction, it must be produced by reactions far more simple than those preceding — the oxidation or hydration of well-defined compounds, for instance. This is what I have endeavored to realize.
Phosphorescence by oxidation is exceptional. That of phosphorus is very probably not due to a simple oxidation only, as I have shown elsewhere.
I have obtained other phosphoresences by oxidation, but they border on incandescence as much as on phosphorescence, and constitute perhaps a transition between the two. Such is notably the case with uranium.
Let us take a strip of this metal about 1 mm thick, and place it on a plate heated to about 600° C. It becomes vividly brilliant. Withdraw it with pincers, and it continues to sine for two or three minutes, which would not happen if it were a simple phenomenon of incandescence that was in question. The same operation may be repeated several times, but the duration of the luminosity of the metal becomes shorter each time, and finally — no doubt when it is entirely oxidized — heat no longer produces any effect upon it.
The only clearly defined reaction which allowed me to obtain phosphorescence is hydration. The best-defined cases are those of sulfates of quinine and cinchonine. Heated to 150° C, these salts lose part of their water, and after shining for an instant again become completely dark. When they have thus lost all their phosphorescence, they are allowed to cool; they become at once hydrated by contact with the air, again become phosphorescent, and at the same time radioactive. I shall not dwell upon this experiment, the details of which I have given in The Evolution of Matter. By reason of its considerable theoretical importance from the point of view of the causes of radioactivity, it has been the object of important papers abroad. The exactness of my observations has thus been clearly confirmed.
It is possible, in the preceding experiment, to render the employment of heat completely useless. It is sufficient to mix quinine sulfate in a bottle with a little anhydrous phosphoric acid which at once dehydrates it. It is hydrated anew by simply breathing on its surface. Persons not previously informed of the theory of the operation are always much surprised when shown a body which becomes phosphorescent when breathed upon.
Quinine sulfate is, of course, not the only compound which possesses the property of becoming phosphorescent by hydration. I have found a whole series of bodies offering the same phenomenon. Such, especially, are ordinary magnesia, commercial calcium sulfate, and hydrated alumina.
These only differ from quinine sulfate by becoming phosphorescent by dehydration alone, instead of phosphorescing, like the first, both by hydration and by dehydration. Further, as these bodies become dehydrated slowly and with difficulty, they have to be heated to nearly 500 C for the phenomenon to be well marked.
Phosphorescence is very easy to observe with calcium sulfate and especially with ordinary magnesia, which becomes very luminous when dehydrating. This is less apparent with alumina.
If, after heating these compounds for some minutes until the phosphorescence is extinct, they are allowed to cool in the dark and then heated again, they again shine. But the luminosity is much greater if their hydration has been rendered more complete by moistening them with a little water before heating them. As soon as the heat is sufficient to produce dehydration, they become very phosphorescent.
The phosphorescence thus obtained can be repeated indefinitely on the same body by simply moistening it the moment it is dried by the heat. This is not at all the case, as we have seen, with bodies made phosphorescent by a simple rise in temperature. Cooled and then heated again, these last no longer become phosphorescent unless in the meantime they have been exposed to the light.
Salts of radium lose, as I have shown in my earlier work, their phosphorescence when hydrated. The very active ones regain it at once after having been dehydrated by heat, those slightly active, only some days after their dessication.
4. Phosphorescence of Living Beings
The phosphorescence of living beings has for a long time been considered as a rather rare phenomenon manifested by a very small number of animals and vegetables.
It has been observed, however, from remote antiquity. Besides the “sea of fire” necessarily known to all navigators by the presence of infusoria, the ancient authors have mentioned the phosphorescence of certain marine animals. Pliny pointed out that the liquid from the pholads renders luminous in the dark the lips and hands of those who eat this mollusk. Fisher relates, in his Manual de Conchologie, that Reaumur “has noted that fragments of these beings remain luminous after their separation from the body, and that when dried, they are able to emit light anew when moistened”.
Until recent years the number of phosphorescent animals known was somewhat restricted. No one could have suspected that the depths of the vast oceans, so long inaccessible, where reigned eternal night, were inhabited by innumerable luminous beings. Since suitable instruments have permitted have permitted the study of the inhabitants of seas at depths of several thousand meters, a complete new world has been revealed.
It than became known that the bottom of the sea was covered with veritable forests of phosphorescent polyps; that the smallest as well as the most bulky of the beings inhabiting these dark depths often possessed organs enabling them to light themselves through the abysses in which they live.
The phosphorescent organs of the marine animals reveal very different dispositions. Some are placed in different parts of the body; others in the eye itself, or above it, and it is quite correctly that the latter have been compared to bicycle lamps. Dr. Richard, curator of the collections of the Prince of Monaco, showed me a whole series of phosphorescent marine animals, and notably large fishes, possessing on each side of the body veritable lamps which they can mask at will. All these beings have been the subject of numerous studies, almost exclusively of an anatomical nature.
In addition to the phosphorescent beings which people the depths of the sea, there should be quoted the luminous bacteria which appear on the bodies of fish after their death and before their decomposition. These bacteria can be cultivated very easily by the classic processes. An excellent culture can be made with ordinary water containing about 3% sea salt and 1% asparagine. It is with analogous products that were obtained the bottles sold by the name of “Living Light” at the Paris Exhibition of 1900. I have prepared some similar ones by simply scraping with a knife blade the scales of a herring and introducing the scrapings into bottles of the above liquid. At the end of 24 hours they possess a luminosity which lasts for 3 or 4 days.
Notwithstanding various researches we have not succeeded in determining the chemical bodies which produce this phosphorescence. We only know that it is a phenomenon which can survive the death of the animal. Carus had already seen that the luminous organs of the Italian lamprey, when dried and powdered, regained their lost phosphorescence on simply being moistened.
These phenomena of the phosphorescence of living beings are certainly due to chemical actions requiring the presence of air and water. When these two elements are suppressed it disappears. It has been shown above that for certain well-defined bodies hydration is always accompanied by phosphorescence.
The spectrum of the phosphorescence of living beings seems to present a few variations differing with the animals. It impresses the photographic plate very rapidly. I have been able to reproduce negatives with two minutes of exposure by taking as the source of light, fish which have become phosphorescent after death by the development of luminous bacteria.
The phosphorescence of living beings presents, in its effects, close analogies to the phosphorescence produced by the various bodies we have already studied. It differs form them, in its causes, by being generated neither by light nor by heat. By its effects as well as by its causes, it is near akin to the phosphorescence by chemical reaction examined above.
5. The Phosphorescence of Gases
The study of electric discharges in gases demonstrates the part played by electricity in the production of phosphorescence. The various causes studies above regarding this phenomenon, such as light and heat, act perhaps by indirectly exciting electric manifestations capable of producing phosphorescence.
In the phosphorescence of gases the action of electricity is perfectly clear, since it alone can render them luminous. The highest temperatures, even when a whole meter of gas is heated, only gives them a hardly perceptible luminosity; whereas, if they are introduced in a rarified state into a tube through which an electric current is passed, they become greatly luminous. It is even sufficient to place the tube containing the rarified gas in the neighborhood of a high frequency resonator, or, again, to subject it to the action of electric waves. With tubes of helium, the luminosity is brilliant enough for it to be used to detect these last.
The phosphorescence obtained by the electrification of gases is relatively bright, but up to late years there seemed no hope of rendering it intense enough for lighting purposes.
The remarkable discovery of the mercury lamp has shown that lighting by phosphorescence was practicable, and proves once more to what a degree incandescence is independent of phosphorescence.
It will be remembered that this lamp consists of a long glass rube furnished with electrodes — one of iron, the other of mercury. If, after having made a vacuum in this tube, these two electrodes are connected to a source of electricity, the traces of gas and mercury vapor that it contains become brightly phosphorescent. In this luminosity there is evidently no phenomenon of incandescence, seeing that the temperature is only about 135° C — that is to say, about much less than that necessary to render a body incandescent. The flame of a simple candle, as we know, has a temperature of about 1700° C.
The spectrum of the phosphorescence of gases, such as that given by the mercury tube, contains very few red and infrared rays, although it has an output much higher than that of the ordinary incandescent light. To obtain this last there must first be produced an enormous quantity of invisible, and therefore useless, caloric rays. Our present process of artificial lighting is barbarous, as has been recognized for sometime. While the ideal method would be to produce only the visible and not the invisible part of the spectrum, a gas flame or the electric arc contains only 1 percent of useful rays, 99 percent of the energy produced manifesting itself in the form of dark heat. With the phosphorescence of the mercury lamp, about 40 percent seems to serve for lighting. The output of the phosphorescent animals, such as the glowworm, is higher still, since nearly the whole of the energy expended is transformed into visible light. Phosphorescence will probably be the artificial light of the future.
It is not for this reason alone necessary to pursue the study of the phosphorescence of gases. I have always suggested that it was too costly for my modest researches, because I am persuaded that its thorough knowledge will open up new vistas on the greatest problems of physics and astronomy.
Phosphorescence by electrical action gives, then, to atoms the same radiating properties as does heat. Intra-atomic energy ought to play some part in the production of this phenomenon, for the gases in the tubes described are strongly ionized — that is to say, dissociated by the passage of the current.
It is probably to the influence of electric actions analogous to those of which the effects have just been shown that certain stars, composed, as their spectrum shows, of gas, owe their luminous brilliancy. Since gaseous bodies cannot shine by incandescence, as we have seen, other causes than heat must be sought for the light of many stars.
But is some stars are luminous simply by electric actions similar to those studied, it would follow that the stars to which one attributes an enormous heat, may be on the contrary at a temperature relatively low. It is perhaps only at a certain phase of their existence that they shine by incandescence.
Chapter IV – The Causes of Phosphorescence
1. Phosphorescence as a Manifestation of Intra-Atomic Energy
Phosphorescence represents the transformation into light of various energies of which only a few are known. In the case of gases we have seen that electric energy is the sole possible cause of the phosphorescence. In that due to the action of light and of heat, and of chemical reactions, other energies intervene, the mode of action of which is still undetermined.
Since very different causes — light, shock or impact, and x-rays — may produce phosphorescence in the same substance, there is ground for believing that these very different excitants act by provoking the manifestation of reactions, which, if not identical, are at least of the same order. The old theory which considered phosphorescent bodies as simply restoring the light absorbed, as a sponge restores the water it has imbibed, is no longer tenable at the present day.
The phenomenon of phosphorescence seems linked with that of the dissociation of matter, and with the liberation of energies which accompany this dissociation. The link is evident as regard the phosphorescence of gases, since the latter only become phosphorescent under the influence of electrical actions, which are always accompanied by ionization — that is to say, by the disintegration of their atoms.
As regards other forms of phosphorescence, the connection with dissociation is far less evident. It appears, however, at least probable in the case of phosphorescence by light, when we remember that light energetically dissociates matter, and that it is just the radiations producing this dissociation which are the most apt to produce phosphorescence.
The tendency to consider phosphorescence as one of the consequences of the dissociation of atoms is beginning to take shape among physicists. De Heen and, after him, Lenard, have recently arrived at the conclusion that light acts by provoking the emission of negative ions issuing from the atom, and capable of returning to it by oscillation.
To these movement of the elements of the dissociated atom should be due the radiation in the ether producing phosphorescence. Its light, of the same nature as that of incandescent bodies, differs from it by the absence of any elevation of temperature, which amply justifies the name of cold light sometimes given to it. It is possible that the rays of light, electricity, and the different causes of phosphorescence bring the intra-atomic energy immediately into play without its having to first pass through molecular movements, as in the case of heat.
It is not strictly proven, moreover, that cold light is not in reality quite as hot as that generated by incandescence. If phosphorescence is a very superficial atomic phenomenon, it may be that the elevated temperature accompanying it is not appreciable, Crookes has observed that by exposing diamonds to the cathode bombardment, their surface is transformed into graphite — which implies, according to Moissan, a temperature of 3600 C — and yet the deeper strata of the diamond experience no notable elevation of temperature. The tube in which it is enclosed is very little heated. The same author has likewise observed that the surface of silver plate can be thus brought to a red heat, while the temperature of the whole mass of metal is hardly raised, notwithstanding the high conductivity of silver for heat. I must remark, however, that after rendering phosphorescent large masses of certain sulfides, spread out so as to have only a thickness of some hundredths of a millimeter, and afterwards collecting the mass in a flask, its temperature was not appreciably raised.
The above consideration on the intra-atomic origins of phosphorescence only constitute the suggestion of an explanation; the complete solution of the problem is still far off.
It is of importance to first thoroughly determine the reactions producing phosphorescence. They are of a special order, for which the laws of the early chemistry are of no service. How, in fact, could be have conceived that reactions bringing into play significant quantities of energy as, for example, the very slight hydration of a salt of quinine, could act on so stable a structure as an atom, and at the same time generate both radioactivity and phosphorescence.
Without being able to entirely elucidate such phenomena, one can at least grasp its possibility by bearing in mind the notion, repeated referred to in the book, that the atom, notwithstanding its stability, may become unstable when we bring to bear upon it a suitable reagent. It then behaves somewhat like a tuning fork, which the most intense noise is powerless to shake, while a slight sound of suitable period will cause it to vibrate. A thin pencil of uv light will thus dissociate without difficulty the atoms of a block of steel capable of withstanding the most violent shocks. When a salt of quinine becomes very phosphorescent and radioactive solely by the addition of a thousandth part of its weight in water-vapor, we have simply — without, however, clearly knowing how — excited reactions giving to the atoms of this body the instability which precedes dissociation.
2. Special Character of the Chemical Reactions Giving to Bodies the Aptitude for Phosphorescence
What has been said will enable us to catch a glimpse of the reason why bodies, never phosphorescent under the influence of light when pure, acquire this property when we add to them a few hundred-thousandth parts of their weight — that is to say, almost imponderable traces — of foreign bodies.
The sulfides of the alkaline earths and natural minerals are never phosphorescent in a state of purity. Certain bodies considered as very pure, such as the fluorite used to make lenses, crystallized apatite, etc., are, however, phosphorescent, but their pure state is only apparent. They always contain traces of foreign bodies. So does the diamond, as chemical analysis of it shows.
It is the special combinations due to the presence of these foreign bodies which give to certain chemical compounds the aptitude for phosphorescence. Once formed, these combinations present some curious characteristics. Very movable, as is proved by the rapidity with which the different rays of light cause phosphorescence to appear or disappear, they nevertheless withstand energetic causes of destruction, since it takes 15 hours of calcinations at red heat to deprive certain substances of their aptitude for phosphorescence. This calcinations in no wise acts by eliminating anything, since the regeneration of the combination and the aptitude for phosphorescence which ensues are obtained by passing induction sparks through the calcined bodies. Heat has not therefore eliminated any foreign body, but has simply destroyed the chemical combinations capable of being accompanied by phosphorescence, which reform under the influence of the electric spark.
The foreign bodies capable of causing phosphorescence must always be present in infinitesimal proportions. Different substances may be substituted for one another to produce effects, if not identical, at all events analogous. M. Mourelo has shown that, to give strontium sulfide the property of becoming phosphorescent by heat, it has only to be calcined with a ten-thousandth part of a salt of manganese or of bismuth. Similar observations have been made with regard to the other sulfides.
The early experiments of Becquerel prove that the slightest variation in the composition of the bodies in the mixture have an influence on their phosphorescence. Marble, chalk, Iceland spar, bodies chemically identical, since they are composed of calcium carbonate, when dissolved in nitric acid give calcium carbonates which we might suppose to be identical. They are not, however; since, by calcining with sulfur the calcium carbonates of various sources we obtain sulfides of calcium of which the phosphorescence may be yellow, green, or violet. The carbonates of lime employed have thus retained infinitesimal traces of foreign bodies varying with their origin.
One of the most singular characteristics of the chemical combinations capable of giving to bodies the aptitude for phosphorescence by light is, as is said above, an extreme mobility — that is to say, the faculty of being formed, and of being destroyed indefinitely, in an almost simultaneous fashion. Certain radiations produce phosphorescence in zinc sulfide in one-tenth of a second, and others destroy it in the same space of time. Different animals, such as the glow-worm, likewise possess this property of causing their phosphorescence to appear and disappear instantaneously.
This notion of chemical reaction capable of being produced in the heart of perfectly solid bodies, such as diamonds, fluorite, and sulfides of alkaline earths, is evidently still outside the range of classic ideas.
These last hardly admit, according to the adage of the early chemists, that bodies can act on one another save in a state of solution; nor do they admit combination in proportions neither simple nor definite. Chemical science, moreover, has no knowledge of combinations which can almost instantaneously be made, unmade, and remade indefinitely under such slight influences as a ray of light.
Of what do these combinations consist where one of the elements is in an infinitely small proportion relatively to the other?
We know by all that has been said that the combinations which render phosphorescence possible realize the following conditions: (1) The bodies, the addition of which produces combinations accompanied by phosphorescence when excited by light or by heat, ought to be added in very small proportions; (2) the combinations formed are mobile and capable of being regenerated, since they are destroyed and remade in a small fraction of a second; (3) these combinations, so rapidly destroyed and so rapidly regenerated, are intimately connected with the action of the temperatures, which, when it is raised, destroys them very quickly, and very slowly when it is sufficiently low. The phosphorescent body emits for months invisible radiations. The return of the combination to its primitive state is completely stopped at a still lower temperature, and the body preserves it phosphorescence indefinitely until its temperature is raised.
Apart from phosphorescent bodies we do not know any chemical combinations able to realize these various conditions, and simple mixtures certainly do not do so. We are therefore obliged to admit that we have to do with an utterly unknown order of chemical reactions. They will probably remain unknown for a long time, because their extreme instability and the ease with which they are destroyed and regenerated protect them against all processes of chemical analysis in present use. To determine the bodies present is of no use, since it is the combinations formed by them which we want to grasp.
The problem is further complicated, because the foreign bodies, the combinations whereof induces the aptitude for phosphorescence, seem only to act by giving instability to the atom so as to allow it to liberate the energies it contains.
We are hardly in reality beginning to suspect the causes of phosphorescence, but that which we catch a glimpse of enables us to feel by anticipation that it will constitute one of the most important chapters of chemistry, and will surely be linked with the history, as yet hardly outlined, of the dissociation of matter.